PFAS
PFAS stands for poly- and perfluoroalkyl substances and loosely refers to organic compounds containing a smaller or larger chain with some or all of C-H replaced with C-F. PFAS can be sorted into severeal subclasses. Some examples of common subclasses are perfluoro- sulfonic acids, carboxylic acids, phosphonic/phosphinic acids, sulfonamides, and alcohols.
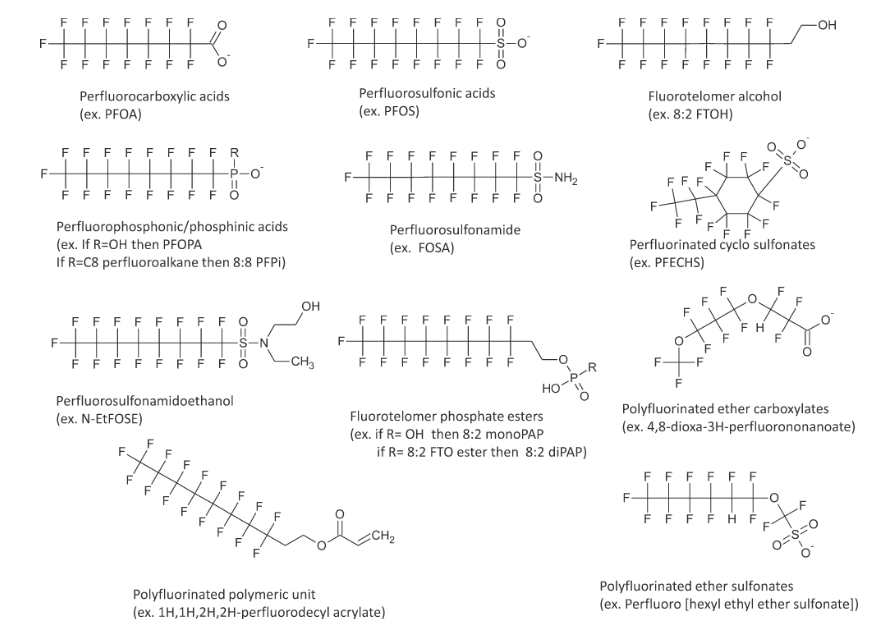
Figure 1. Generic structures for polyfluorinated compounds. The n = 8 linear carbon structures are shown for many of these examples, but n = 4 to 14 linear and/or branched carbon units are generally possible. Image reproduced from Lindstrom et al. (Environ. Sci. Technol., 2011.)
Uses
Contrary to what one may be excused for believing, PFAS compounds were not originally intended to pollute people and the planet. They share some unique features that give them their useful properties and also causes problems when they are not contained and become pollutants. First, the C-F bond is unusally strong, making (the highly fluorinated moiety of) PFAS stable to heat, many reducing and oxidizing conditions, processing by microorganisms, etc. Further, PFAS compounds have both hydrophobic and lipophobic properties. An unusal property that can be visualized as a three-phase system with water and organic solvent and likely stems from the extremely low polarizability of the fluorine atom and corresponding per/polyfluorinated compounds. The stability, water and fat repelling properties are why PFAS compounds are used in food packaging, frying pans, DWR-coated clothing, and fire-fighting foam among many other applications.
Problems
The problems for humans and nature stems from the same properties: they accumulate in (human) tissue due to hydrophobicity and enzymatic stability that make the clearance from the body extremely slow. Once thought inert and safe to health, PFAS compounds have now been shown to cause various diseases, e.g., cancer and thyroid disease, when they accumulate in the body.
Analogously in nature, these so-called "forever chemicals" accumulate in organs and tissues in organisms at sea and land. Often taken up by microorganism and then moving up the food chain, creating a biomagnification on top of the bioaccumulation.
Of interest in our current PFAS project are PFAS in fire-fighting foam that contaminate soil and ground water. The contaminated water in this study is leachate from soil from fire fighting practice areas at airports.
PFAS removal strategies and the rotating bed reactor
Strategies for PFAS remediation of contaminated soil can broadly be classified into i) immobilization ii) mobilization iii) removal/degradation. Point ii) and iii) are commonly combined, i.e., liquid that is used to wash soil from PFAS is further treated to remove and degrade PFAS. This is the direct procedure for contaminated lakes, sea, and ground water.
Point i) involves restricting the hydrodynamic movement in the soil by in situ barriers or mixing with Portland cement or transport to crates. It can also involve adding additives to the soil, e.g., activated carbon, that bind stronger than the soil to PFAS and prevents high levels of PFAS in soil leachate. However, it can be argued that additives do not contain any PFAS but simply extend the leakage.
Point ii) consists of washing/removing the PFAS from the soil. Water is the most obvious and arguable most reasonable liquid for washing. Water with a range of additives has been tried, such as salts, acids, bases, and detergents. Organic solvents and supercritical carbon dioxide has also been attempted. Desorption by heating is another strategy for removing PFAS from soil and other solids. A complication is that the there are 4000+ known PFAS compounds which have different physicochemical properties, notably solubility and vapor pressure in this context. Thus, some are easier than others to remove. Generally, the smaller PFAS compounds are more soluble and have higher vapor pressure.
Point iii) finally commonly involves capturing and concentrating PFAS from water and storing or destructing the concentrate. Optionally, PFAS could be destructed without prior concentration in contaminated water. Also, attempts has been made to destruct PFAS still in the soil.
For capturing and concentrating PFAS from water, there are some more or less established methods in use. Recently in the news, soil remediation by plant uptake has been discussed. This involves planting in contaminated soil and harvesting vegetable material that has taken up some of the PFAS that is mobilized in the water in the soil. The smaller PFAS are more mobile in the soil and are preferentially taken up, which is useful since the methods discussed below are more effective against the larger PFAS compounds. The vegetable material is subsequently burned in a facility suited for PFAS contaminated material. For removing PFAS from leachate and other contaminated waters, two major concepts are the SAF method and capture on adsorbents. The SAF method firstly involves airating the water to make the PFAS float to the surface as a foam, which can be skimmed off. As mentioned, the method is most effective for the larger PFAS compounds. Finally, adsorbents come in many variants and applications. The major classes are granulated activated carbon and ion exchange materials. Activated carbon is general-purpose for organic compounds, which is both its strength and weakness. Spent activated carbon can be sent back to the manufacturer to be re-activated but is commonly single-use (by the customer/user). Synthetic ion exchange (IEX) beads have the benefit that they can be designed to be somewhat selective and that they are less friable than granualted activated carbon. IEX beads are naturally only effective against ionic PFAS compounds such as perfluoroalkyl sulfonic acids, carboxylic acids, phosphonic acids and more. Both single-use and regenerable (by the customer/user) IEX beads are available. IEX beads have traditionally been used in column systems. A long established and working technology, which however requires a large hardware installment and CAPEX. SpinChem is offering an alternative for applying adsorbents to liquids with a different working principle to column technology and a few unique advantages. More on this below.
As for the destruction of PFAS compounds, the mentioned stability of the compounds makes this a difficult tasks. High temperature incineration at suitable facilities can be used for vegetable material and PFAS concentrate. Alternatively, for PFAS in water, strongly oxidizing conditions may be used. Oxidants such as persulfate is an option for concentrate, for dilute water solutions ozone and Fenton conditions may be more suitable.
RBR implementation
While IEX resin in column systems are well-established, rotating bed reactors have their own set of advantages that make them a strong option in many cases:
- flexibility in deployment, RBR:s can be used in most types of vessels or containments. Operation can be either batch-style in a large body of contaminated water or semi-continuous (stop-flow), i.e., by processing a larger body of water in a rapid series of smaller portions.
- lower CAPEX vs. columns
- small footprint
- The points above make it a viable option for ad hoc deployment when the need arises, e.g., as an add-on to water treatment plants.
- can be made portable for on-location processing such as pump-and-treat.
- freedom to chose, change and combine adsorbents. While granulated activated carbons work well for many pollutants including many PFAS compounds, current IEX resins tailored for PFAS are arguable even more attractive by offering better selectivity, high capacity and the option to be regenerated by the user. We are happy to discuss good adsorbent options.
Additionally, the RBR operation can be automated which further improves utility and efficiency.